Fellowship
Optimisation and validation of 3D models of progressive human lung fibrosis
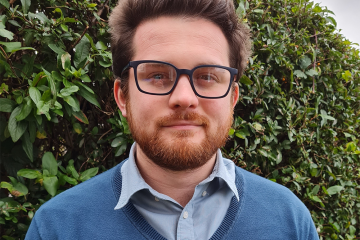
At a glance
Completed
Award date
April 2021 - March 2023
Grant amount
£114,352
Principal investigator
Dr Joseph Bell
Institute
University of Southampton
R
- Replacement
Read the abstract
View the grant profile on GtR