PhD Studentship
Modelling of thrombus dynamics in a microfluidic 'vasculature'
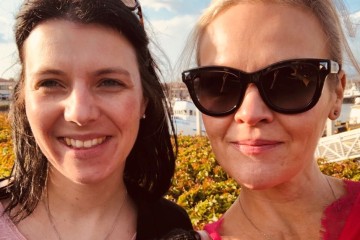
At a glance
In progress
Award date
July 2022 - June 2025
Grant amount
£90,000
Principal investigator
Dr Nicola Mutch
Co-investigator(s)
Institute
University of Aberdeen
R
- Replacement
Read the abstract
View the grant profile on GtR